This article is joint work with Dr. John Newhook, professor and Dean of Engineering at Dalhousie University in Halifax.
Over the past year we have been asked numerous times by athletes and coaches alike if they should brush faster – that is, with a higher stroke rate – than what they are doing currently, with the underlying assumption that brushing more quickly will lead to improved brushing performance. A possible reason for these questions is the success of several of the women’s teams from Asia over the past few seasons, who from observation do brush a bit more quickly than their typical Canadian competitors. However, as with many things brushing any answer is dependent on a number of variables and trade-offs, and in no small way is also dependent on the force profile(s) of the particular players. In this article, we’ll take a look at the science in the available literature, coupled with our own experiences, and try to supply some guidance to answer this question.
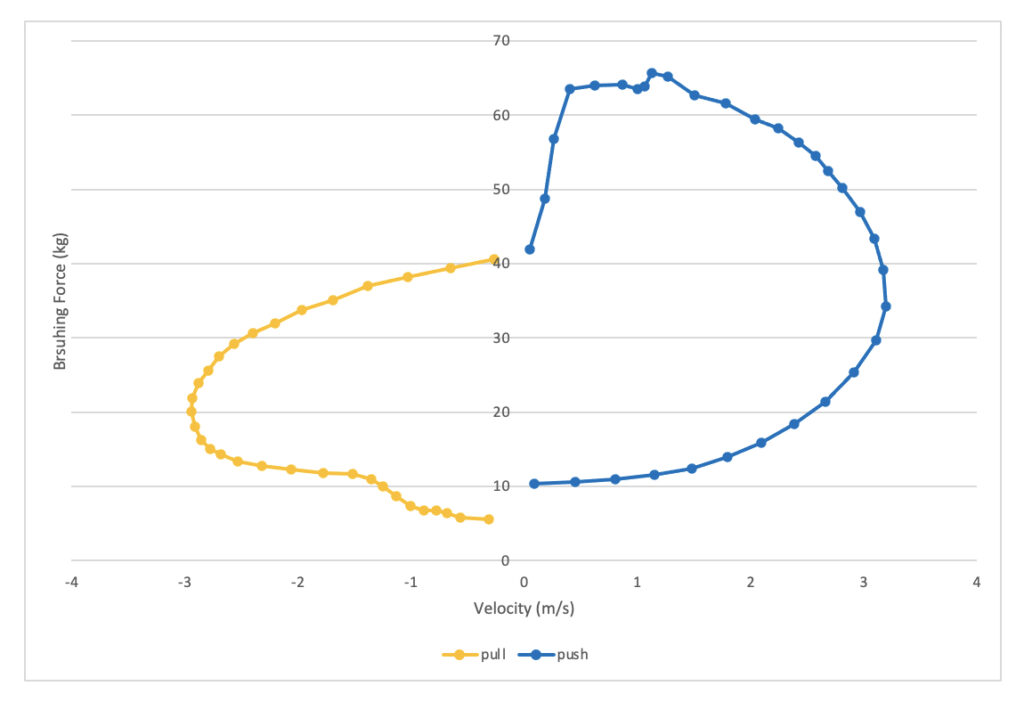
Figure 1. Vertical force, stated in kilograms, relative to brush head velocity during a single brush stroke. Captured in the Biodynamics, Ergonomics and Neuroscience (BEN) Lab at Dalhousie University in Halifax, using an AMTI BP400600-100 force plate with a 4450 Newton capacity.
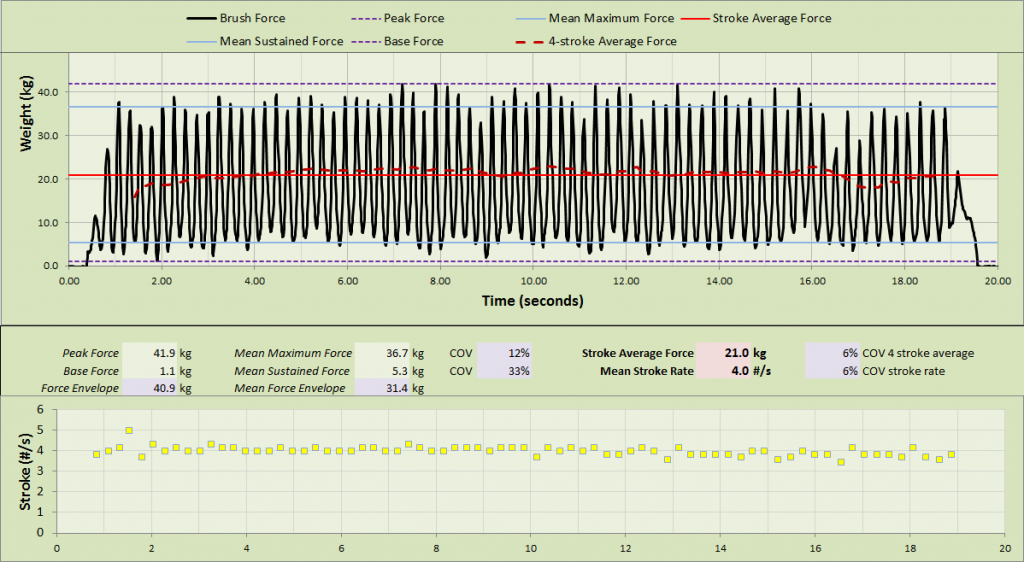
Figure 2. Typical results from a high-performance female university athlete, with a mean (vertical) force of 21.0 kg (47% of her body weight) and a mean stroke rate of 4.0 Hz. Note the low 6% COV (co-efficient of variance) of the athlete’s stroke rate over the 19-second bout.
Results from the literature
As an answer to the question “Should one brush faster” we are, at this time of writing (December 2024), unaware of any published or unpublished study that analyzes on-ice brushing performance through comparing slower and faster stroke rates for either increasing carry or managing stone trajectories. One of the reasons that undertaking such a study would be difficult is because it is hard to isolate stroke rate from two other tightly related variables: stroke displacement and force profile.
However, there are published studies (see the References below) that have looked at brushing variables such as stroke displacement that can give an indication of the depth of the trade-offs involved. One thing to remember is that “corner sweeping” and brushing in a “snowplough” position, now commonplace brushing tactics, were illegal prior to the 2016 imposition of standard Oxford 55 D520 mustard yellow nylon brush pad fabric in competitive play. The majority of past results do not provide an athlete’s angle of attack, but it would be unlikely that a snowplough brushing technique would be included in the results [1,2,5].
Stroke displacement

A. A single brush stroke with a displacement of 190 mm captured in the laboratory using an AMTI BP400600-100 force plate.
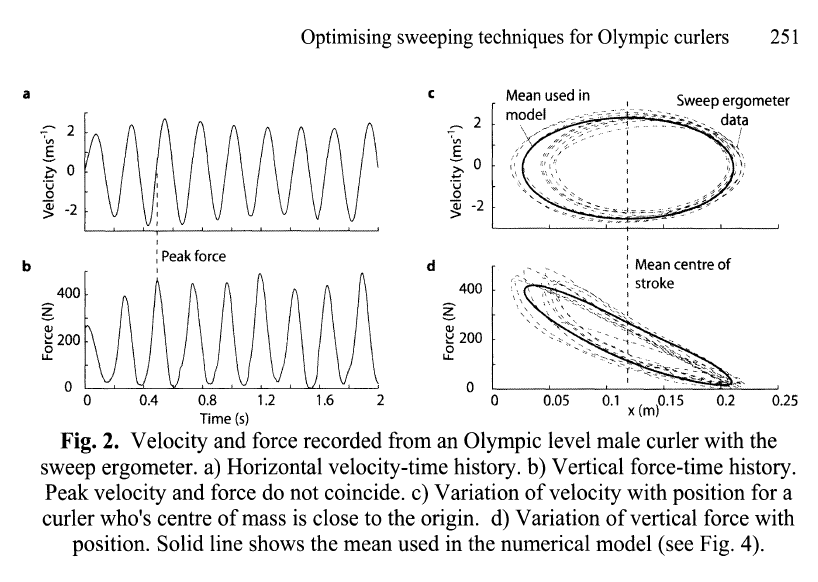
B. Sample stroke data from Marmo et al. [6].

C. Experimental data from Bradley [1].
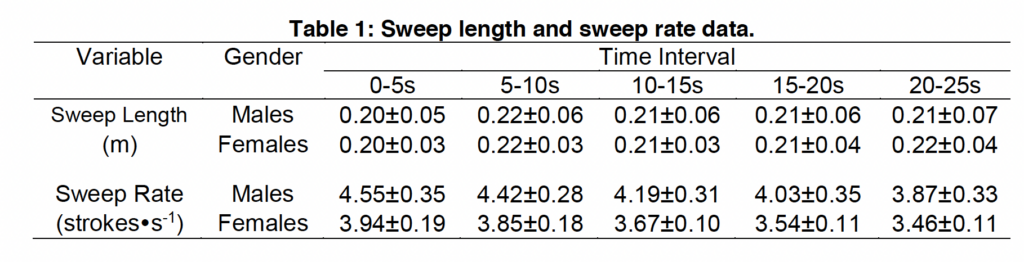
D. Average sweep data statistics from Kivi et al. [5].
Figure 3 – Experimental data for stroke displacement, from four different sources.
Neither a CurlSmart instrumented brush nor the commercially-available instrumented brushes currently available directly measure stroke displacement (or stroke length). In [5], Kivi and his colleagues at Lakehead University used video analysis via a Dartfish video system to analyze stroke displacement for 16 competitors (8 male, 8 female) and determined an average stroke displacement of around 21 cm (see Figure 3(D) above). In [6], Marmo presents brushing characteristics for a single Olympic athlete and from the graph in Figure 3(B) above it appears that the typical displacement is approximately 19 cm. Marmo’s result is in good agreement with our experience in measuring athletes in the BEN laboratory at Dalhousie (see Figure 3(A) above as but one example).
In contrast, Bradley’s [1] results give a displacement of approximately half of Kivi’s values, 10.7 cm versus 20-22 cm. These results are surprising, however the average vertical force values presented in his paper are equally surprisingly in that they are exceedingly low, and in no way reflect the vertical force achieved by the vast majority of U18 competitive athletes, or those in more advanced LTCD stages, at least in our experience. This is something that we have already commented on previously.
In our experience in measuring athletes in the lab, a 20 cm stroke displacement is typical across athletes of both genders and different LTCD stages. Since the nominal diameter of the running band of a curling stone is 13.3 cm it makes sense that the displacement of any stroke that crosses the path of the stone should be at least that long, if not longer to ensure that no portion of the ice in front of the running band is missed. One possible technique that can be used to increase one’s stroke rate, however, is to reduce the displacement of each stroke so that the travel time of each push or pull component is shortened; but that may mean that ice coverage in front of the stone will be reduced. The other method, of course, is to increase the velocity of the brush head during each portion of the stroke so that more strokes can be accomplished in the same amount of time, with no impact on stroke displacement. Note, however, that with a typical displacement of 20 cm and a typical stroke rate of 4.3 Hz the brush head is already travelling at a peak velocity of 3 m/sec (see Figure 1 above), higher than the rate described by Marmo et al.
One reason for maintaining a stroke displacement of approximately 20cm is that the horizontal force generated during the push portion of the stroke continues to be higher – nearly double – than that which is achieved during the pull portion of the stroke (Figure 4 at right). Horizontal force produced during the stroke has an impact on both warming and pebble abrasion, though precisely to what extent remains a research problem.
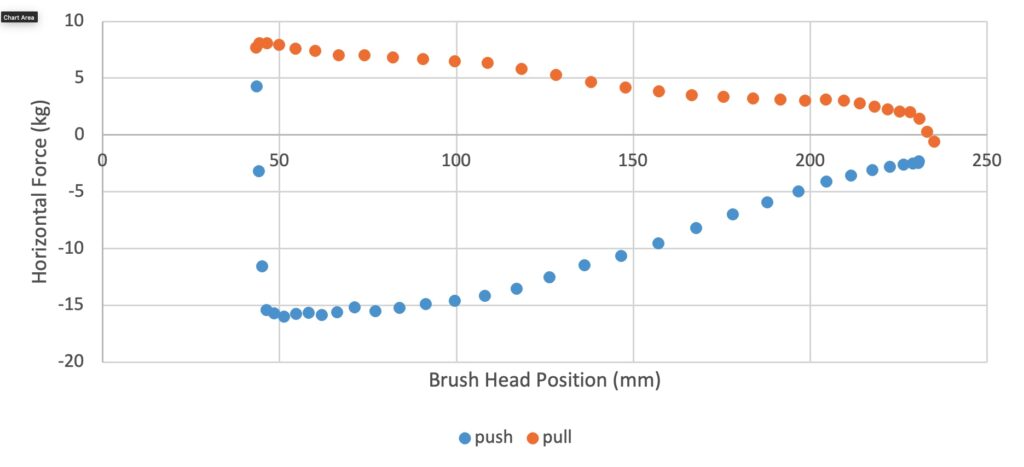
Figure 4. Brush head position versus horizontal force, in kg, captured on an AMTI BP400600-100 force plate. Note that the force values are relative to an origin on the force plate, so forces in one direction are negative, and positive in the other direction of the stroke.
Stroke rate
In contrast to stroke displacement, stroke rate does tend to vary by gender, Long-Term Curler Development (LTCD) stage, and often by the angle of attack and/or brushing stance (open, closed, or snowplough). There are a number of reasons for this, including the physical characteristics of the athletes [4] and their biomechanical position when brushing. Commonly, in human performance there is an inverse relationship between speed and force, and in our experience we frequently encounter athletes who brush (slightly) more quickly than their peers, but whose force values are below average.
Nevertheless, in the data captured by one of the authors (Paulley), comprising 571 unique athletes and approximately 2000 measured bouts using a CurlSmart instrumented brush, the stroke rate for male and female athletes across all LTCD stages falls between 3.7 and 5.1 Hz (full strokes – push and pull – per second) with very few outliers outside that range. For top-tier competitive male players in the Train to Compete, Learn to Excel, or Train to Excel LTCD stages the mean stroke rate is approximately 4.3 Hz with a very small standard deviation (0.2), in good agreement with Bradley’s results (see Figure 3(C) above). For top-tier female athletes the average is slightly higher at 4.4 Hz but the standard deviation is much larger, reflecting a broader spread of values across the different LTCD stages. This result differs significantly from Kivi’s results (see Figure 3(D) above). Unfortunately it is difficult to place Kivi’s results in context because their study does not include force profiles for each of the tested athletes. Moreover, in our experience, fit competitive athletes tend not to exhibit significant decreases in stroke rate through an end-to-end bout; the stroke rate tends to remain fairly constant throughout (see Figure 2 above for one example).
One rationale for increasing one’s stroke rate is to generate more overlapping strokes on the ice surface to further increase ice temperature, mentioned by both Marmo [6] and Bradley [1]. However, for a low angle of attack (brushing across the face of the stone) a single player will not be able to generate overlapping strokes even at draw weight until the rock begins to slow. At release, a stone for a draw-weight shot is moving at approximately 2.1 metres/second, or 210 cm. With a typical brush head of 20 cm in width, a player could cover the ice in front of the stone just once if they could brush at 5 Hz (possible); to cover each portion of the ice surface twice would require brushing at 10 Hz (rather unlikely). However, once the stone begins to slow at the far hog line, the stone’s velocity will fall to 0.8-1.0 m/sec and overlapping strokes can be achieved even at typical stroke rates of 4.3-4.4 Hz. Moreover, a simple mechanism to increase ice coverage is simply to utilize two brushers, rather than one.
Summary
In our experience with coaching athletes, it is challenging for athletes to increase their stroke rate by greater than, say, 10% without suffering from either (a) a shorter stroke displacement or (b) reducing their force output on the brush. It is commonplace today for players to only “clean” a stone for the first half of a shot, and then brush as necessary after the stone has travelled half the distance to the house. In addition, because of the narrow range of stroke rates that we see in top-level players, we continue to focus on coaching optimal biomechanics for force generation rather than focus on speed. Once an athlete achieves above-average force, then we can consider increasing the athlete’s stroke rate as long there remains room and is not detrimental to stroke displacement or their force profile. However, as stated earlier, an on-ice study that clearly illustrates the characteristics of the stroke rate/force profile trade-off is still needed.
References
[1] Bradley, J. L. (2009). The sports science of curling: a practical review. Journal of Sports Science and Medicine 8, pp. 495-500.
[2] Jenkyn, T. (2010). Report of findings to the Canadian Curling Association from the project titled: “Investigation of the mechanics and biomechanics of curling using real-time motion analysis”. University of Western Ontario, 28 pages. Confidential.
[3] Kim, T.-W., S.-C. Lee, S.-K. Kil, S.-H. Choi, and Y.-G. Song (2021). A Case Study on Curling Stone and Sweeping Effect According to Sweeping Conditions. International Journal of Environmental Research and Public Health 18, pp. 883. DOI: https://doi.org/10.3390/ijerph18020833
[4] Kivi, D., T. Auld, C. Leon-Carlyle, and D. Witiluk (2013). Physical and Physiological Assessment of Competitive Junior Curlers. Unpublished manuscript. Available at https://curlnoca.ca/wp-content/uploads/2013/06/Curling-Fitness-Assessment-Study.pdf
[5] Kivi, D., T. Auld, and C. Zerpa (2014). Sweep length versus sweep rate analysis in competitive male and female curlers. In Proceedings, International Society of Biomechanics in Sports, pp. 606–609.
[6] Marmo, B. A., M-P Buckingham, and J. R. Blackford (2006). Optimising sweeping techniques for Olympic curlers. The Engineering of Sport 6(3), pp. 249-254. Abstract: Sports Engineering 9(4), pp. 249.